The Specially-Designed Elements
of Life
There
is a mind and purpose behind the universe. There
are hints of that divine presence in how abstract
mathematics can penetrate the universe's secrets,
which suggests that a rational mind created
the world. Nature is fined tuned to allow life
and consciousness to emerge.
John Polkinghorne, British Physicist 84
Up to this point we have been examining how
all the physical balances of the universe in
which we live have been specially designed so
that we can live. We have seen how the general
structure of this universe, the location of
Earth in it, and factors such as air, light,
and water have been precisely designed to have
exactly the attributes we require. In addition
to all this however, we also need to take a
look at the elements that make up our bodies.
These chemical elements, the building-blocks
from which our hands, eyes, hair, and organs
as well as all the living things-plants and
animals-that are our sources of food have been
specially designed to serve the exact purposes
that they do.
The physicist
Robert E. D. Clark refers to the special and
superior design in the building-blocks of life
when he says: "As if the Creator has given us
a kit of prefabricated parts ready made for
the work in hand."85
The most important of these building-blocks
is carbon.
The Design in Carbon
In previous chapters we described
the extraordinary process by which carbon, the
element that occupies the sixth position in
the periodic table, was produced in the hearts
of the huge stars called "red giants". We also
saw how, having discovered this wonderful process,
Fred Hoyle was moved to say that "the laws of
nuclear physics have been deliberately designed
with regard to the consequences they produce
inside the stars."86
When we examine carbon more closely, we can
see that not just the physical formation of
this element but also its chemical properties
were deliberately arranged to be what they are.
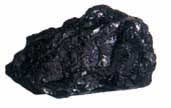
One natural form of pure carbon is
graphite. This element however is able to
form an extraordinary range of different
substances when it combines with the atoms
of other elements. The main structure of
the human body is the result of the different
chemical bonds that carbon is capable of
entering into. |
Pure carbon occurs naturally in two forms:
graphite and diamonds. Carbon however also enters
into compounds with many other elements and
the result is many different kinds of substances.
In particular, the incredibly varied range of
organic materials of life-the membrane of a
cell and the bark of a tree, the lens of an
eye and the horn of a deer, the white of an
egg and the poison of a snake-are all made up
of carbon-based compounds. Carbon, combined
with hydrogen, oxygen, and nitrogen in many
different quantities and geometric arrangements,
results in a vast assortment of materials with
vastly different properties.
Some carbon compounds' molecules consist of
just a few atoms; others contain thousands or
even millions. Furthermore, no other element
is as versatile as carbon is in forming molecules
with such durability and stability. To quote
David Burnie in his book Life:
Carbon is
a very unusual element. Without the presence
of carbon and its unusual properties, it is
unlikely that there would be life on Earth.87
Concerning carbon, the British chemist Nevil
Sidgwick writes in Chemical Elements and their
Compounds:
Carbon is
unique among the elements in the number and
variety of the compounds which it can form.
Over a quarter of a million have already been
isolated and described, but this gives a very
imperfect idea of its powers, since it is the
basis of all forms of living matter.88
For reasons of both physics
and chemistry, it is impossible for life to
be based on any element other than carbon. At
one time, silicon was proposed as another element
on which life might be based. We now know however
that this conjecture is impossible. To quote
Sidgwick again:
We know enough now to be sure
that the idea of a world in which silicon should
take the place of carbon as the basis of life
is impossible…89
Covalent Bonds
The chemical bonds that carbon enters into
when forming organic compounds are called "covalent
bonds". A covalent bond is said to occur when
two atoms share their electrons.
The electrons of an atom occupy specific orbital
shells that are centered around the nucleus.
The orbit closest to the nucleus can be occupied
by no more than two electrons. In the next orbit
a maximum of eight electrons is possible. In
the third orbit, there can be up to eighteen.
The number of electrons continues to increase
with the addition of more orbits. Now an interesting
aspect of this scheme is that atoms seem to
"want" to complete the number of electrons in
their orbital shells. Oxygen, for example, has
six electrons in its second (and outermost)
orbit, and this makes it "eager" to enter into
combinations with other atoms that will supply
the two more electrons that are needed to increase
this number to eight. (Why atoms behave this
way is a question that is unanswered. But it's
a good thing they do: because if they didn't,
life wouldn't be possible.)
Covalent bonds are the result of this tendency
of atoms to complete their orbital shells. Two
or more atoms can often make up the shortfall
in their orbits by sharing electrons with one
another. A good example is the water molecule
(H2O), whose building-blocks (two
hydrogen atoms and one oxygen atom) form a covalent
bond. In this compound, oxygen completes the
number of electrons in its second orbit to eight
by sharing the two electrons (one each) in the
orbital shells of the two hydrogen atoms; in
the same way, the hydrogen atoms each "borrow"
one electron from oxygen to complete their own
shells.
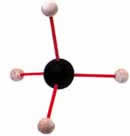
The structure of methane: Four hydrogen
atoms share one electron each with a single
carbon atom. |
Carbon is very good at forming covalent bonds
with other atoms (including carbon atoms) from
which an enormous number of different compounds
can be made. One of the simplest of these compounds
is methane: a common gas that is formed from
the covalent bonding of four hydrogen atoms
and one carbon atom. With only six electrons,
carbon's outer orbital shell is short of the
eight that it needs by four, rather than two
as is the case with oxygen, and for this reason,
four hydrogen atoms are needed to complete it.
We said that carbon was especially versatile
in forming bonds with other atoms and this versatility
makes an enormous number of different compounds
possible. The class of compounds formed exclusively
from carbon and hydrogen are called "hydrocarbons".
This is a huge family of compounds that includes
natural gas, liquid petroleum, kerosene, and
lubricating oils. Hydrocarbons like ethylene
and propylene are the "bedrock" on which the
modern petrochemical industry has been erected.
Hydrocarbons like benzene, toluene, and turpentine
are familiar to anyone who's worked with paints.
The naphthalene that protects our clothes from
moths is another hydrocarbon. With the addition
of chlorine in their composition, some hydrocarbons
become anesthetics; with the addition of fluorine,
we have Freon, a gas that is widely used in
refrigeration.
There is another important class of compounds
in which carbon, hydrogen, and oxygen form covalent
bonds with one another. In this family we find
alcohols like ethanol and propanol, ketones,
aldehydes, and fatty acids among many, many
other substances. Another group of compounds
composed of carbon, hydrogen, and oxygen are
sugars, including glucose and fructose.
The cellulose that makes up the skeleton of
wood and the raw material for paper is a carbohydrate.
So is vinegar. So is beeswax and formic acid.
Each one of the incredibly rich panoply of substances
and materials that occur naturally in our world
is "nothing more" than a different arrangement
of carbon, hydrogen, and oxygen linked together
by covalent bonds.
Olive oil, meat, and brown sugar: Everything
we eat is made up of different arrangements
of hydrogen, oxygen, and carbon with the
addition of other atoms such as nitrogen.
|
When carbon, hydrogen, oxygen, and nitrogen
form such bonds, the result is a class of molecules
that is the foundation and structure of life
itself: the amino acids that make up proteins.
The nucleotides that make up DNA are also molecules
formed from carbon, hydrogen, oxygen, and nitrogen.
In short, the covalent bonds that the carbon
atom is capable of entering into are vital for
the existence of life. Were hydrogen, carbon,
nitrogen, and oxygen not so "eager" to share
electrons with one another, life would indeed
be impossible.
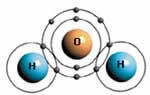
WATER AND METHANE: TWO DIFFERENT EXAMPLES
OF COVALENT BONDS
In the water molecule (above), there is
a covalent bond between the two hydrogen
atoms and the one oxygen atom. In the methane
molecule seen above on the right, four hydrogen
atoms form covalent bonds with a single
carbon atom. |
The thing that makes it possible for carbon
to form these bonds is a property that chemists
call "metastability", the characteristic of
having only a slight margin of stability. The
biochemist J. B. S. Haldane describes metastability
thus:
A metastable
molecule means one that can liberate free energy
by a transformation, but is stable enough to
last a long time unless it is activated by heat,
radiation, or union with a catalyst.90
What this somewhat technical definition means
is that carbon has a rather unique structure,
thanks to which, it is quite easy for it to
enter into covalent bonds under normal conditions.
But it is precisely here that the situation
starts to become curious because carbon is metastable
only within a very narrow range of temperatures.
Specifically, carbon compounds become very unstable
when the temperature goes over 100°C.
This fact is so commonplace in our everyday
lives that most of us take it for granted. When
we cook meat for example, what we're really
doing is changing the structure of its carbon
compounds. But there's a point here that we
should note: The cooked meat has become completely
"dead"; that is, its chemical structure is different
from what it had when it was part of a living
organism. Indeed most carbon compounds become
"denatured" at temperatures above 100°C: the
majority of vitamins for example simply fall
apart at that temperature; sugars also undergo
structural changes and lose some of their nutritional
value; and at around 150°C, carbon compounds
will start to burn.
In other words, if carbon atoms are to enter
into covalent bonds with other atoms and if
the resulting compounds are to remain stable,
the ambient temperature must not go over 100°C.
The lower boundary on the other hand is around
0°C: if the temperature drops too much below
that, organic biochemistry becomes impossible.
In the case of other compounds, this is generally
not the situation. Most inorganic compounds
are not metastable; that is, their stability
is not greatly affected by changes in temperature.
To see this let's do an experiment. Stick a
piece of meat on the end of a long, thin piece
of metal such as iron and heat the two together
over a fire. As the temperature grows hotter,
the meat will darken and eventually burn long
before much of anything happens to the metal.
The same thing would be true if you substituted
stone or glass for metal. You would have to
increase the heat by many hundreds of degrees
before the structures of such materials began
to change.
By now you certainly will have spotted the
similarity between the temperature range that
is necessary for carbon compounds' covalent
bonds to be established and remain stable and
the range of temperatures that prevails on our
planet. As we have said elsewhere, in the whole
universe, temperatures range from the millions
of degrees in the hearts of stars to absolute
zero (-273.15°C). But Earth, having been created
for humanity to live in, possesses the narrow
temperature range essential for the formation
of the carbon compounds that are the building-blocks
of life.
But the curious "coincidences" do not end here.
The same temperature interval is the only one
in which water remains liquid. As we saw in
the earlier chapter, liquid water is one of
the basic requirements of life and, in order
to remain liquid, it requires precisely the
same temperatures that carbon compounds need
to form and be stable. There is no physical
or natural "law" dictating that this should
be so and under the circumstances, this situation
is evidence that the physical properties of
water and carbon and the conditions of the planet
Earth were created so as to be in harmony with
one another.
Weak Bonds
Covalent bonds are not the only type of chemical
bonding that keeps the compounds of life stable.
There is another and different category of bond
known as "weak bonds".
Such bonds are about twenty times weaker than
covalent bonds, hence their name; but they are
no less crucial to the processes of organic
chemistry. It is thanks to this weak bonding
that the proteins that make up the building-blocks
of living things are able to maintain their
complex and vitally important three-dimensional
structures.
To explain this, we have to talk briefly about
the structure of proteins. Proteins are usually
referred to as a "chain" of amino acids. While
this metaphor is essentially correct, it is
also incomplete. It's incomplete because for
most people a "chain of amino acids" conjures
up the mental image of something like a string
of pearls whereas the amino acids that make
up proteins have a three-dimensional structure
more like a tree with leafy branches.
Covalent bonds are what hold the atoms of amino
acids together. Weak bonds are what maintain
the essential three-dimensional structure of
those acids. No proteins could exist without
these weak bonds. And of course without proteins,
there could be no life.
Covalent bonds: The atoms are strongly
linked to one another |
Weak bonds: An organic compound is maintained
in a unique three-dimensional form by
means of weak (non-covalent) bonds (the
broken lines). |
Now the interesting part of this business is
that the temperature range in which weak bonds
are able to perform their function is the same
as the one prevailing on Earth. This is rather
odd because the physical and chemical natures
of covalent bonds versus weak bonds are entirely
different things and independent of one another.
In other words, there's no intrinsic reason
why they should both require the same temperature
range. And yet they do: Both types of bonds
can only be formed and remain stable within
this narrow temperature range. And if they did
not-if covalent bonds required a range of temperatures
wildly different from that of weak bonds, say-then
it would be impossible to construct the complex
three-dimensional structures that proteins require.
Everything that we have seen concerning the
extraordinary chemical properties of the carbon
atom shows that there is an enormous harmony
existing among this element that is the fundamental
building-block of life, the water that is also
vital for life, and the planet Earth that is
the shelter for that life. In Nature's Destiny,
Michael Denton underscores this fitness when
he says:
Out of the
enormous range of temperatures in the cosmos,
there is only one tiny temperature band in which
we have (1) liquid water, (2) a great plenitude
of metastable organic compounds, and (3) weak
bonds for stabilizing the 3-D forms of complex
molecules.91
Among all the heavenly bodies that have ever
been observed, this "tiny temperature band"
exists only on Earth. Moreover it is only on
Earth that the two fundamental building-blocks
of life--carbon and water-are to be found in
such generous supply.
What all this indicates is that the carbon
atom and its extraordinary properties were specially
designed for life and that our planet was specially
created to be a home for carbon-based life-forms
The Design in Oxygen
We have seen how carbon is the most important
building-block of living organisms and how it
was specially designed so as to fulfill that
function. The existence of all carbon-based
life-forms however is contingent upon a second
imperative: energy. Energy is an indispensable
requirement for life.
Green plants get their energy from the sun
through the process of photosynthesis. For the
rest of the living creatures of Earth-and that
includes us-the only source of energy is a process
called "oxidation"-a fancy word for "burning".
The energy of oxygen-breathing organisms is
derived from burning the nourishment that they
get from plants and animals. As you may guess
from the term "oxidation", this burning is a
chemical reaction in which substances are oxidized-that
is, they are combined with oxygen. This is why
oxygen is as vitally important to life as are
carbon and hydrogen.
A generalized formula for "burning" (oxidation)
looks like this:
Carbon compound + oxygen > water + carbon dioxide
+ energy
What this means is that when carbon compounds
and oxygen are combined (under the proper conditions
of course) a reaction takes place that generates
water and carbon dioxide and releases a considerable
amount of energy. This reaction takes place
most readily in hydrocarbons (compounds of hydrogen
and carbon). Glucose (a sugar and also a hydrocarbon)
is what is constantly being burned in your body
to keep it supplied with energy.
Now as it happens, the elements of hydrogen
and carbon that make up hydrocarbons are the
ones most suitable for oxidation to take place.
Among all other atoms, hydrogen combines with
oxygen the most readily and releases the most
energy in the process. If you need a fuel to
burn in oxygen, you can't do better than hydrogen.
From the standpoint of its value as a fuel,
carbon ranks third after hydrogen and boron.
In The Fitness of the Environment, Lawrence
Henderson comments on the extraordinary fitness
that is involved here:
The very chemical
changes, which for so many other reasons seem
to be best fitted to become the processes of
physiology, turn out to be the very ones which
can divert the greatest flood of energy into
the stream of life.92
The Design in Fire (Or
Why You Don't Just Burst Into Flame)
As we've just seen, the fundamental reaction
that releases the energy necessary for the survival
of oxygen-breathing organisms is the oxidation
of hydrocarbons. But this simple fact raises
a troubling question: If our bodies are made
up essentially of hydrocarbons, why aren't they
also oxidized? Putting it another way, why don't
we just go up in flame, like a match that's
been struck?
Our
bodies are constantly in contact with the oxygen
of the air and yet they don't oxidize: they
don't catch fire. Why not?
The reason for this seeming paradox is that,
under normal conditions of temperature and pressure,
the molecular (O2) form of oxygen
has a substantial degree of inertness or "nobility".
(In the sense that chemists use the term, "nobility"
is the reluctance (or inability) of a substance
to enter into chemical reactions with other
substances.) But this raises another questions:
If molecular oxygen is so "noble" as to avoid
incinerating us, how is this same molecule made
to enter into chemical reactions inside our
bodies?
The answer to this question,
which perplexed chemists as early as the mid
19th century, did not become known until the
second half of the 20th century, when biochemical
researchers discovered the existence of enzymes
in the human body whose only function was to
force the O2 in the atmosphere to
enter into chemical reactions. As a result of
a series of extremely complex steps, these enzymes
utilize atoms of iron and copper in our bodies
as catalysts. A catalyst is a substance that
initiates a chemical reaction and allows it
to proceed under different conditions (such
as lower temperature etc) than would otherwise
be possible.93
In other words, there is a very interesting
situation here: Oxygen is what supports oxidation
and combustion and normally one would expect
it to burn us up too. To prevent this, the molecular
O2 form of oxygen that exists in
the atmosphere has been given a strong element
of chemical nobility. That is, it doesn't enter
into reactions easily. But, on the other hand,
our bodies depend upon the oxidizing property
of oxygen for their energy and for that reason,
our cells have been fitted out with an extremely
complex enzyme system that makes this noble
gas extremely reactive.
While we're on the subject
we should also point out that this enzyme system
is a marvellous example of design that no evolutionary
theory holding that life developed as a result
of chance events can ever hope to explain.94
There is yet another precaution
that has been taken to keep our bodies from
burning up: what the British chemist Nevil Sidgwick
calls the "characteristic inertness of carbon".95
What this means is that carbon is not too much
in a hurry either to enter into a reaction with
oxygen under normal pressures and temperatures.
Expressed in the language of chemistry this
may all seem rather arcane, but in fact what
is being said here is something that anyone
who's ever had to light a fireplace full of
huge logs or a coal-burning stove in winter
or start a stubborn barbecue in summer already
knows. In order to get the fire going, you have
to take care of a lot of preliminaries (kindling,
starter, etc) or else suddenly raise the temperature
of the fuel to a very high degree (as with a
blowtorch). But once the fuel starts burning,
the carbon in it enters into the reaction with
oxygen quite rapidly and a great amount of energy
is released. This is why it's so hard to get
a fire going without another source of heat.
But after combustion begins, a great deal of
heat is produced and this can cause other carbon
compounds nearby to catch fire as well and so
the fire spreads.
When we look into this matter more carefully,
we can see that fire itself is a most interesting
example of design. The chemical properties of
oxygen and carbon have been so arranged that
these two elements enter into a reaction with
one another (combustion) only when a great amount
of heat is already present. It's a good thing,
too because if this weren't the case, life on
this planet would be very unpleasant if not
downright impossible. If oxygen and carbon were
even slightly more willing to react with one
another, the spontaneous combustion-self-ignition-of
people, trees, and animals would become a commonplace
event whenever the weather got a little too
warm. Someone walking through a desert for example
might suddenly burst into flame at noon when
the heat was at its most intense; plants and
animals would be exposed to the same risk. Even
if life were possible in such a world, it certainly
wouldn't be much fun.
On the other hand, if carbon and oxygen were
slightly more noble (that is, slightly less
reactive) than they are, it would be much more
difficult to light a fire in this world than
it already is: indeed, it might even be impossible.
And without fire, we not only would have been
unable to keep ourselves warm: it's quite likely
that there would never have been any technological
progress on our planet because that progress
depends upon the ability to work materials such
as metal and without the heat provided by fire,
purifying and working metal is all but impossible.
What all this shows is that the chemical properties
of carbon and oxygen have been arranged so as
to be the most suitable for the needs of mankind.
Concerning this, Michael Denton says:
This curious
unreactivity of the carbon and oxygen atoms
at ambient temperatures, combined with the enormous
energies inherent in their combination once
achieved, is of great adaptive significance
to life on Earth. It is this curious combination
that not only makes available to advanced life
forms the vast energies of oxidation in a controlled
and orderly manner but has also made possible
the controlled use of fire by mankind and allowed
the harnessing of the massive energies of combustion
for the development of technology.96
In other words, both carbon and the oxygen
have been created with properties that are the
most fit for human life. The properties of these
two elements allow us to light a fire and to
make use of fire in the most convenient way
possible. Furthermore, the world is full of
sources of carbon (such as the wood of trees)
that are fit for combustion. All this is an
indication that fire and the materials to start
and sustain it have been specially created to
be fit for human life. In the Qur'an, Allah
speaks to mankind with these words:
He Who produces fire
for you from green trees so that you use them
to light your fires. (Surah Ya-sin: 80)
The Ideal Solubility of
Oxygen
The utilization of oxygen by the body is highly
dependent upon the property of this gas to dissolve
in water. The oxygen that enters our lungs when
we inhale is immediately dissolved into the
blood. The protein called hemoglobin captures
these oxygen molecules and carries them to the
other cells of the body where, thanks to the
special enzyme system described above, the oxygen
is used to oxidize carbon compounds called ATP
to release their energy.
All complex organisms derive their energy in
this way. However the operation of this system
is especially dependent upon the solubility
of oxygen. If oxygen were not sufficiently soluble,
not enough oxygen would enter the bloodstream
and cells would not be able to generate the
energy they require; if oxygen were too soluble
on the other hand, there would be an excess
of oxygen in the blood resulting in a condition
known as oxygen toxicity.
The difference in the water-solubility of different
gases varies by as much as a factor of a million.
That is, the most soluble gas is a million times
more soluble in water than the least soluble
gas is and there are hardly any gases at all
whose solubilities are identical. Carbon dioxide
is about twenty times more soluble in water
than oxygen is for example. Among the vast range
of potential solubilities however, the one possessed
by oxygen is precisely what it needs to be for
it to be fit for human life.
What would happen if the water-solubility rate
of oxygen were different: a little more or a
little less?
Let us take a look at the first situation.
If oxygen were less soluble in water (and thus
also in blood) less oxygen would enter the bloodstream
and the body's cells would be starved of oxygen.
This would make life much more difficult for
metabolically active organisms such as human
beings. No matter how hard you worked at breathing,
you would constantly be faced with the danger
of suffocation because not enough oxygen was
reaching your body's cells.
If the water-solubility of oxygen were higher
on the other hand, you would be confronted by
the threat of oxygen toxicity, mentioned briefly
above. Oxygen is, in fact, a rather dangerous
substance: if an organism gets too much of it,
the result can be fatal. Some of the oxygen
in the blood enters into a chemical reaction
with the blood's water. If the amount of dissolved
oxygen becomes too high, the result is the production
of highly reactive and damaging by-products.
One of the functions of the complex system of
blood enzymes is to prevent this from happening.
But if the amount of dissolved oxygen becomes
too high, the enzymes cannot do their job. As
a result, every breath we take would poison
us a little bit more leading quickly to death.
The chemist Irwin Fridovich comments on this
issue:
All respiring
organisms are caught in a cruel trap. The very
oxygen which supports their lives is toxic to
them and they survive precariously, only by
virtue of elaborate defense mechanisms.97
What saves us from this trap-from being poisoned
by too much oxygen or from being suffocated
by not enough of it-is the fact that oxygen's
solubility and the body's complex enzymatic
system have been carefully designed and created
to be what they need to be. To put it more explicitly,
Allah has created not only the air we breathe
but also the systems that make it possible to
use that air in perfect harmony with one another.
The Other Elements
Carbon and oxygen of course are not the only
elements that have been deliberately designed
to make life possible. Elements like hydrogen
and nitrogen, which make up a large part of
the bodies of living things, also possess attributes
that make life possible. In fact, there appears
not to be a single element in the periodic table
that does not fulfill some sort of function
in support of life.
In the basic periodic table
there are ninety-two elements ranging from hydrogen
(the lightest) to uranium (the heaviest). (There
are of course other elements beyond uranium
but these do not occur naturally and have all
been created under laboratory conditions. None
of them are stable.) Of this ninety-two, twenty-five
are directly necessary for life and of those,
just eleven-hydrogen, carbon, oxygen, nitrogen,
sodium, magnesium, phosphorus, sulfur, chlorine,
potassium, and calcium-make up some 99% of the
body weight of nearly all living things. The
other fourteen elements (vanadium, chromium,
manganese, iron, cobalt, nickel, copper, zinc,
molybdenum, boron, silicon, selenium, fluorine,
and iodine) are present in living organisms
only in very small amounts but even these have
vitally important functions. Three elements-arsenic,
tin, and tungsten-are to be found in some living
things where they perform functions that are
not completely understood. Three more elements-bromine,
strontium, and barium-are known to be present
in most organisms, but their functions are still
a mystery.98
This broad spectrum encompasses atoms from
each of the different series of the periodic
table, whose elements are grouped according
to the attributes of their atoms. What this
indicates is that all of the element groups
of the periodic table are necessary, in one
way or another, for life. In The Biological
Chemistry of the Elements, J. J. R Frausto da
Silva and R. J. P Williams have this to say:
The biological elements seem
to have been selected from practically all groups
and subgroups of the periodic table... and this
means that practically all kinds of chemical
properties are associated with life processes
within the limits imposed by environmental constraints.99
Even the heavy, radioactive
elements at the end of the periodic table have
been marshaled in the service of human life.
In Nature's Destiny, Michael Denton describes
in detail the essential role that these radioactive
elements, such as uranium, play in the formation
of the earth's geological structure. Naturally
occurring radioactivity is closely associated
with the fact that the earth's core is able
to retain its heat. That heat is what keeps
the core, which consists of iron and nickel,
liquid. This liquid core is the source of the
earth's magnetic field which, as we have seen
elsewhere, helps shield the planet from dangerous
radiation and particles from space while performing
other functions as well. Even the inert gases
and elements such as the rare-earth metals,
none of which seem to be involved in the support
of life, are apparently there because of the
demands of ensuring that the range of naturally-occurring
elements would extend as far as uranium.100
In short, it is safe to say that all the elements
whose existence we know of serve some function
in human life. Not one of them is either superfluous
or purposeless. This situation is further evidence
that the universe was created by Allah for mankind.
Conclusion
Every physical and chemical property of the
universe that we have examined turns out to
be exactly what it needs to be in order for
life to exist. And yet in this book we have
only scratched the surface of the overwhelming
evidence of this fact. No matter how deeply
you delve the details or broaden the search,
this general observation remains true: In every
detail of the universe, there is a purpose that
serves human life and each detail is perfectly
designed, balanced, and harmonized to achieve
that purpose.
Certainly this is proof of the existence of
a superior creator who brought this universe
into being for this purpose. Whatever property
of matter we may examine, we behold in it the
infinite knowledge, wisdom, and power of Allah,
Who created it from nothingness. Every thing
bows to His will and that is why each and every
thing is in perfect harmony with everything
else.
This is the conclusion that 20th-century science
has at last reached. And yet, it is only a recognition
of a fact that was imparted to mankind in the
Qur'an over fourteen centuries ago: Allah has
created every detail of the universe to reveal
the perfection of His own creation:
Blessed be He who has
the Kingdom in His Hand! He has power over all
things. He who created the seven heavens in
layers. You will not find any flaw in the creation
of the All-Merciful. Look again-do you see any
gaps? Then look again and again. Your sight
will return to you dazzled and exhausted. (Surat
al-Mulk: 1-4) |